Молекулярно-генетическая характеристика экспортера ароматических аминокислот YddG Escherichia Coli
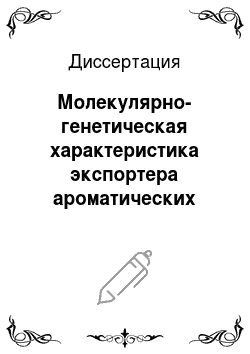
Диссертация
Escherichia coli, в силу ее генетической' и биохимической изученности и из-за разработанности методов манипулирования с ее геномом, — является удобным микроорганизмом для рационального конструирования на ее основе штаммов-продуцентов различных биологически активных соединений и, прежде всего, аминокислот. Для последних можно не изобретать новый путь биосинтеза: клетка Е. coli синтезирует для… Читать ещё >
Список литературы
- Abramson J., Smirnova I., Kasho V., Verner G., Kaback H.R., Iwata S. (2003) Structure and mechanism of the lactose permease of Escherichia coli. Science 301: 610−615.
- Akabas M.H., Stauffer D.A., Xu M., Karlin A. (1992) Acetylcholine receptor channel structure probed in cysteine-substitution mutants. Science. 258: 307−310.
- AkiyamaY., Ito K. (1993) Folding and assembly of bacterial’alkaline phosphatase in vitro and in vivo. J. Biol. Chem. 268: 8146−8150.
- Aleshin V.V., Zakataeva N. P, Livshits V.A. (1999) A new family of amino-acid-efflux proteins. Trends Biochem. Sci. 24: 133−135.
- Alexeyev M.F., Winkler H.H. (1999) Membrane topology of the Rickettsia prowazekii ATP/ADP translocase revealed by novel dual pho-lac reporters. J.Mol.Biol. 285:1503−13.
- Andersson H., von Heijne G. (1993) Sec dependent and sec independent assembly of E. coli inner membrane proteins: the topological rules depend on chain length. EMBO J. 12: 683−91.
- Belitsky B.R., Gustafsson M.C., Sonenshein A.L., von Wachenfeldt C. (1997) An Lrp-like gene of Bacillus subtilis involved in branched-chain amino acid transport. J. Bacteriol. 179: 5548−5557.
- Bellmann A., Vrljic M., Patek M., Sahm H., Kramer R., Eggeling L. (2001) Expression control and specificity of the basic amino acid exporter LysE of Corynebacterium glutamicum. Microbiology. 147: 1765−1774.
- Bendtsen J.D., Nielsen H., von Hcijne G., Brunak S. (2004) Improved prediction of signal peptides: SignalP 3.0. J. Mol. Biol. 340: 783−795.
- Benoit S., Abaibou H., Mandrand-Berthelot M.A. (1998) Topological analysis of the aerobic membrane-bound formate dehydrogenase of Escherichia coli. J. Bacteriol. 180: 6625−34.
- Bernsel A., Viklund II., Hennerdal A., Elofsson A. (2009) TOPCONS: Consensus prediction of membrane protein topology. Nucl. Acids Res. 37: W465−468.
- Blobel G. (1980) Intracellular protein topogenesis. Proc. Natl. Acad. Sei. USA. 77: 14 961 500.
- Bogdanov M., Heacock P.N., Dowhan W. (2002) A polytopic membrane protein displays a reversible topology dependent on membrane lipid composition. EMBO J. 21: 2107−16.
- Bogdanov M., Zhang W., Xie J., Dowhan W. (2005) Transmembrane protein topology mapping by the substituted cysteine accessibility method (SCAM™): application to lipid-specific membrane protein topogenesis. Methods. 36: 148−171.
- Booth I.R., Louis P." (1999) Managing hypoosmotic stress: Aquaporins and mechanosensitive channels in Escherichia coli. Curr. Opin. Microbiol. 2: 166−169.
- Bomemann T., Jockel J., Rodnina M.V., Wintermeyer W. (2008) Signal sequence-independent membrane targeting of ribosomes containing short nascent peptides within the exit tunnel. Nat. Struct. Mol. Biol. 15: 494−499.
- Bowers C.W., Lau F., Silhavy T.J. (2003) Secretion of LamB-LacZ by the signal recognition particle pathway of Escherichia coli. J. Bacteriol. 185: 5697−5705.
- Bradford M.M. (1976) A rapid and sensitive method for quantification of microgram quantities of protein utilizing principle of protein-dye binding. Anal.Biochem. 72:248−54.
- Broer S., Kramer R. (1991a) Lysine excretion by Corynebacterium glutamicum. 1. Identification of a specific secretion carrier system. Eur. J. Biochem. 202: 131−135.
- Broer S., Kramer R. (1991b) Lysine excretion by Corynebacterium glutamicum. 2. Energetics and mechanism of the transport system. Eur. J. Biochem. 202: 137−143.
- Broome-Smith J.K., Tadayyon M., Zhang Y. (1990) ?-Lactamase as a probe of membrane protein assembly and protein export. Mol, Microbiol. 4: 1637−1644.
- Bulaj G., Kortemme T., Goldenberg D.P. (1998) Ionization-reactivity relationships for cysteine thiols in polypeptides. Biochemistry. 37: 8965−8972.
- Busby S., Ebright R.H. (1999) Transcription activation by catabolite activator protein (CAP). J.Mol.Biol. 293: 199−213.
- Calvo J.M., Matthews R.G. (1994) The leucine-responsive regulatory protein, a global regulator of metabolism in Escherichia coli. Microbiol. Rev. 58: 466−490.
- Celebi N., Yi L., Facey S.J., Kuhn A., Dalbey R.E. (2006) Membrane biogenesis of subunit II cytochrome bo’oxidase: contrasting requirements for insertion of N-terminal and C-terminal domains. J. Mol. Biol. 357: 1428−1436.
- Chalfie M. (1995) Green fluorescent protein. Photochem. Photobiol. 4: 651−6.
- Chalfie M., Tu Y., Euskirchen G., Ward W.W., Prasher D.C. (1994) Green fluorescent protein as a marker for gene expression. Science. 263: 802−5.
- Chang C.N., Kuang W.J., Chen E.Y. (1986) Nucleotide sequence of the alkaline phosphatase gene of Escherichia coli. Gene. 44: 121−125.
- Chang X.B., Hou Y.X., Jensen T.J., Riordan J.R. (1994) Mapping of cystic fibrosis transmembrane conductance regulator membrane topology by glycosylation site insertion. J. Biol. Chem. 269: 18 572−18 575.
- Charbit A., Ronco J., Michel V., Werts C., Hofnung -M. (1991) Permissive sites and, topology of an outer membrane protein with a reporter epitope. J. Bacteriol. 173: 262−75.
- Chen M., Samuelson J.C., Jiang F., Muller M., Kuhn A., Dalbey R.E. (2002) Direct interaction of YidC with the Sec independent Pf3 coat protein during its membrane protein insertion. J. Biol. Chem. 277: 7670−7675.
- Chen M., Xie K., Yuan J., Yi L., Facey S.J., Pradel N., Wu L.F., Kuhn A., Dalbey R.E. (2005) Involvement of SecDF and YidC in the membrane insertion of M13 procoat mutants. Biochemistry. 44: 10 741−10 749.
- Claros M.G., von Heijne G. (1994) TopPredll: an improved software for membrane protein structure predictions. Comput. Appl. Biosci. 10: 685−686.
- Csonka L.N., Epstein W. (1996) Osmoregulation. In: Escherichia coli’and Salmonella. Cellular and molecular biology / Eds. F.C. Neidhardt, R. Curtiss III, J.L. Ingraham. Washington: ASM Press, 1996. P.1210−1224.
- Cui Y., Wang Q., Stormo G.D., Calvo J.M. (1995) A consensus sequence for binding of Lrp to DNA. J. Bacteriol. 177: 4872−4880.
- Da?ler T., Maier T., Winterhalter C., Bock A. (2000) Identification of a major facilitator protein from Escherichia coli involved in efflux of metabolites of the cystein pathway. Mol. Microbiol. 36: 1101−1112.
- Dalbey R.E., Chen M. (2004) Sec-translocase mediated membrane protein biogenesis. Biochimica et Biophysica Acta. 1694: 37−53.
- Deitermann S., Sprie G.S., Koch H.-G. (2005) A dual function for SecA in the assembly of single spanning membrane proteins in Escherichia coli. J. Biol. Chem. 280: 39 077−85.
- DeLisa M.P., Wu C.-F., Wang L., Valdes J.J., Bentley W.E. (2001) DNA microarray-based identification of genes controlled by autoinducer 2-stimulated quorum sensing in Escherichia coli. J. Bacteriol. 183: 5239−5247.
- Derman A.I., Prinz W.A., Belin D., Beckwith J. (1993) Mutations that allow disulfide bond formation in the cytoplasm of Escherichia coli. Science. 262: 1744—1747.
- Diaz-Mejia J.J., Babu M., Emili A. (2009) Computational and experimental approaches to chart the Escherichia coli cell envelope-associated proteome and interactome. FEMS Microbiol. Rev. 33: 66−97.
- Donahue J.L., Okpodu C.M., Cramer C.L. (1997) Responses of antioxidants to paraquat in pea leaves. Plants physiol. 113: 249−257.
- Doroshenko V., Airich L., Vitushkina M., Kolokolova A., Livshits V., Mashko S. (2007) YddG from Escherichia coli promotes export of aromatic amino acids. FEMS Lett. 275: 312−318.
- Duong F., Wickner W. (1997) Distinct catalytic roles of the SecYE, SecG and SecDFyaiC subunits of preprotein translocase holoenzyme. EMBO J. 16: 2756−68.
- Duquesne K., Sturgis J.N. (2010) Membrane protein solubilization. Methods Mol. Biol. 601:205−17.
- Ebbighausen H., Weil B., Kramer R. (1989) Transport of branched-chain amino acids in Corynebacterium glutamicum. Arch. Microbiol. 151: 238−244.
- Eggeling L., Sahm H. (2003) New ubiquitous translocators: amino acid export by Corynebacterium glutamicum and Escherichia coli. Arch. Microbiol. 180: 155−160.
- Eisenberg D., Weiss R.M., Terwilliger T.C. (1982) The helical hydrophobic moment: a measure of the amphiphilicity of a helix. Nature. 299: 371−374.
- Elsliger M.A., Wachter R.M., Hanson G.T., Kallio K., Remington S.J. (1999) Structural and spectral response of green fluorescent protein variants to changes in pH. Biochemistry. 38: 5296−5301.
- Erdmann A., Weil B., Kramer R. (1993) Lysine secretion by wild-type Corynebacterium glutamicum triggered by dipeptide uptake. J. Gen. Microbiol. 139: 3115−3122.
- Facey S.J., Kulm A. (2010) Biogenesis of bacterial inner membrane proteins. Cell. Mol. Life Sei. 67: 2343−62.
- Facey S.J., Neugebauer S.A., Krauss S., Kuhn A. (2007) The mechanosensitive channel protein MscL is targeted by the SRP to the novel YidC membrane insertion pathway of Escherichia coli. J. Mol. Biol. 365: 995−1004.
- Feilmeier B.J., Iseminger G., Schroeder D., Webber H., Phillips G.J. (2000) Green fruorescent protein functions as a reporter for protein localization in Escherichia coli. J. Bacteriol. 182: 4068−4076.
- Franke I., Resch A., Da? ler T., Maier T., Bock A. (2003) YfiK from Escherichia coli promotes export of O-acetylserine and cysteine. J. Bacteriol. 2003. 185: 1161−1166.
- Frillingos S., Sahin-Toth M., Wu J., Kaback H.R. (1998) Cys-scanning mutagenesis: a novel approach to structure-function relationships in polytopic membrane proteins. FASEBJ. 12: 1281−1299.
- Froderberg L., Houben E., Samuelson J.C., Chen M., Park S.-K., Phillips G.J., Dalbey R., Luirink J., de Gier J.-W.L. (2003) Versatility of inner membrane protein biogenesis in Escherichia coli. Mol. Microbiol. 47: 1015−1027.
- Geller B.L., Wickner W. (1985) M13 procoat inserts into liposomes in the absence of other membrane proteins. J. Biol. Chem. 260: 13 281−13 285.
- Gollub E., Zalkin H., Sprinson D.B. (1970) Assay for 3-deoxy-D-arabino-heptulosonic acid 7-phosphate synthase. Methods Enzymol. 17: 349−350.
- Gurskaya N.G., Savitsky A.P., Yanushevich Y.G., Lukyanov S.A., Lukyanov K.A. (2001) Color transitions in coral’s fluorescent proteins by site-directed mutagenesis. BMC Biochemistry. 2: 6.
- Gurskaya N.G., Fradkov A.F., Terskikh A., Matz M.V., Labas Y.A., Martynov V.l., Yanushevich Y.G., Lukyanov K.A., Lukyanov S.A. (2001a) GFP-like chromoproteins as a source of far-red fluorescent proteins. FEBS Lett. 507: 16−20.
- Gyaneshwar P., Paliy O., McAuliffe J., J ones A., Jordan M.I., Kustu S. (2005) Lessons from Escherichia coli genes similarly regulated in response to nitrogen and sulfur limitation. Proc. Natl. Acad. Sci. USA. 102: 3453−3458.
- Haney S.A., Platko J., Oxender D.L., Calvo J.M. (1992) Lrp, a leucine-responsive protein, regulates branched-chain amino acid transport genes in Escherichia coli. J. Bacteriol. 174: 108−115.
- Hatzixanthis K., Palmer T., Sargent F. (2003) A subset of bacterial inner membrane proteins integrated by the twin-arginine translocase. Mol. Microbiol. 49: 1377−1390.
- Heddle C., Mazaleyrat S.L. (2007) Development of a screening platform for directed evolution using the reef coral fluorescent protein ZsGreen as a solubility reporter. Prot. Engin. Design Selection. 20: 327−337.
- Heim R., Cubitt A.B., Tsien R.Y. (1995) Improved green fluorescence. Nature. 373: 663 664.
- Heim R., Prasher D.C., Tsien R.Y. (1994) Wavelength mutations and posttranslational autoxidation of green fluorescent protein. Proc. Natl. Acad. Sci. USA. 91: 12 501−12 504.
- Hermann T., Kramer R. (1996) Mechanism and regulation of isoleucine excretion in Coiynebacterium glutamicum. Appl. Environ. Microbiol. 62: 3238−3244.
- Herskovits A.A., Bochkareva E.S., Bibi E. (2000) New prospects in studying the bacterial signal recognition particle pathway. Mol. Microbiol. 38: 927−939.
- Hirokawa T., Boon-Chieng S., Mitaku’S. (1998) SOSUI: classification and secondary structure prediction system for membrane proteins. Bioinformatics. 14: 378−379.
- Hoffman C.S., Wright A. (1985) Fusions of secreted proteins to alkaline phosphatase: an approach for studying protein secretion. Proc. Natl. Acad. Sci. USA. 82: 5107−5111.
- Hofmann K., Stoffel W. (1993) Tmbase a database of membrane spanning proteins segments. Biol. Chem. Hoppe-Seyler. 347: 166.
- Hopp T.P., Woods K.R. (1981) Prediction of protein1 antigenic determinants from amino acid sequences. Proc. Natl. Acad. Sci. USA. 78: 3824−3828.
- Houben E.N., ten Hagen-Jongman C.M., Brunner J., Oudega B., Luirink J. (2004) The two membrane segments of leader peptidase partition one by one into the lipid bilayer via a Sec/YidC interface. EMBO Rep. 5: 970−975.
- Ikeda M., Arai M., Lao D., Shimizu T. (2001) Transmembrane topology prediction methods: a re-assessment and improvement by a consensus method using a dataset of experimentally-characterized transmembrane topologies. In Silico Biol. 2: 1−15:
- Jager H" Birkenhager R., Stalz W.-D., Altendorf K., Deckers-IIebestreit G. (1998) Topology of subunit a of the Escherichia coli ATP synthase. Eur.J.Biochem. 251:122−32.I
- Jewell J.E., Orwiek J., Liu J., Miller K.W. (1999) Functional importance and local1 environments of the cysteines in the tetracycline resistance protein encoded by plasmid pBR322. J. Bacteriol. 181: 1689−1693.
- Jones D.T. (1998) Do transmembrane protein superfolds exist? FEBS. Lett. 423:281−285.
- Jones D.T. (2007) Improving the accuracy of transmembrane protein topology prediction using evolutionary information. Bioinformatics. 23: 538−544.
- Kali L., Krogh A., Sonnhammer E.L. (2004)A combined transmembrane topology and signal peptide prediction method. J. Mol: Biol. 338: 1027−1036.
- Kail L., Krogh A., Sonnhammer E.L. (2005) An HMM posterior decoder for sequence feature prediction that includes homology information. Bioinformatics 21. Suppl. l: i251— 1257.
- Kali L., Krogh A., Sonnhammer E.L. (2007) Advantages of combined transmembrane topology and signal peptide prediction the Phobius web server. Nucleic Acids Res. 35: W429−32.
- Karlin A., Akabas M.H. (1998) Substituted-cysteine accessibility method. Methods Enzymol. 293: 123−145.
- Keenan R.J., Freymann D.M., Stroud R.M., Walter P. (2001) The signal recognition particle. Annu. Rev. Biochem. 70: 755−775.
- Kennerknecht N., Sahm H., Yen M-R., Patek M., Saier M.H. Jr., Eggeling L. (2002) Export of L-isoleucine from Corynebacterium glutamicum: a two-gene-encoded member of a new translocator family. J. Bacteriol. 184: 3947−3956.
- Kiefer D., Kuhn A. (2007) YidC as an essential and multiiiinctional component in membrane protein assembly. Int. Rev. Cytol. 259: 113−138.
- Kimura T., Ohnuma M., Sawai T., Yamaguchi A. (1997) Membrane topology of the transposon 10-encoded metal-tetracycline/H+ antiporter as studied by site-directed chemical labeling. J. Biol. Chem. 272: 580−585.
- Klenner C., Yuan J., Dalbey R.E., Kuhn A. (2008) The Pf3 coat protein contacts TM1 and TM3 of YidC during membrane biogenesis. FEBS Lett. 582: 3967−3972.
- Koch H.G., Moser M., Schimz K.L., and Muller M. (2002) The integration of YidC into the cytoplasmic membrane of Escherichia coli requires the signal recognition, particle, SecA and SecYEG. J. Biol. Chem. 277: 5715−5718.
- Koch H.G., Muller M. (2000) Dissecting the translocase and’integrase functions of the Escherichia coli SecYEG translocon. J. Cell. Biol. 150: 689−94.
- Kohler R., Boehringer D., Greber B., Bingel-Erlenmeyer R., Collinson I., Schaffitzel C.", Ban N. (2009) YidC and Oxal form dimeric insertion pores on the translating ribosome. Mol. Cell. 34: 344−353.
- Kol S., Majczak W., Heerlien R., van der Berg J.P., Nouwen N., Driessen A.J.M. (2009) Subunit a of the FiF0 ATP synthase requires YidC and SecYEG for membrane insertion. J. Mol. Biol. 390: 893−901.
- Kramer R. (1994) Secretion of amino acids by bacteria: physiology and mechanism. FEMS Microbiol. Rev. 13: 75−94.
- Krebs M.P., Noorwez S.M., Malhotra R., Kaushal S. (2004) Quality control of integral membrane proteins. Trends Biochem. Sei. 29: 648−55.
- Krogh A., Larsson B., von Heijne G., Sonnhammer E.L.L. (2001) Predicting transmembrane protein topology with a hidden Markov model: application to complete genomes. J. Mol. Biol. 305: 567−580.
- Kuhn A. (2009) From the Sec complex to the membrane insertase YidC. Biol. Chem. 390: 701−706.
- Kummer A.D., Wiehler J., Rehaber H., Kompa C., Steipe B., Michel-Beyerle M.E. (2000) Effects of threonine 203 replacements on excited-state dynamics and fluorescence properties of the green fluorescent protein (GFP). J. Phys. Chem. 104: 4791−4798.
- Kyte J., Doolittle R.F. (1982) A simple method for displaying the hydropathic character of a protein. J. Mol. Biol. 157: 105−132.
- Laemmli U.K. (1970) Cleavage of structural proteins during the assembly of the head of bacteriophage T4. Nature. 227: 680−685.
- Laishram R.S., Gowrishankar J. (2007) Environmental regulation operating at the promoter clearance step of bacterial transcription. Genes Dev. 21: 1258−72.
- Lange R., Hengge-Aronis R. (1991) Identification of a central regulator of stationary-phase gene expression in Escherichia coli. Mol. Microbiol. 5: 49−59.
- Lee C., Li P., Inouye H., Brickman E.R., Beckwith J. (1989) Genetic studies on the inability of ?-galactosidase to be translocated across the Escherichia coli cytoplasmic membrane. J. Bacteriol. 171: 4609−4616.
- Lee P.A., Tullman-Ercek D., Georgiou G. (2006) The bacterial twin-arginine translocation pathway. Annu. Rev. Microbiol. 60: 373−395.
- Lewin B. Genes VIII. Upper Saddler River, NJ 7 458: Pearson Education, 2004. P.283.
- Link A.J., Dereth P., Church G.M. (1997) Methods for generating precise deletions and insertions in the genome of wild-type Escherichia coli: application to open reading frame characterization. J. Bacteriol. 179: 6228−6237.
- Liu X.Y., Matherly L.H. (2002) Analysis of membrane topology of the human reduced folate carrier protein by hemagglutinin epitope insertion and scanning glycosylation insertion mutagenesis. Biochim. Biophys. Acta. 31: 333−342.
- Livshits V.A., Zakataeva N.P., Aleshin V.V., Vitushkina M.V. (2003) Identification and characterization of the new gene rhtA involved in threonine and homoserine efflux in Escherichia coli. Res. Microbiol. 154: 123−135.
- Loo T.W., Clarke D.M. (1995) Membrane topology of a cysteine-less mutant of human P-glycoprotein. J. Biol. Chem. 270: 843−848.
- Luirink J., von Heijne G., Houben E., de Gier J.-W. (2005) Biogenesis of inner membrane proteins in Escherichia coli. Annu. Rev. Microbiol. 59: 329−355.
- Ma J., Katsonouri A., Gennis R.B. (1997) Subunit II of the cytochrome bo3 ubiquinol oxidase from Escherichia coli is a lipoprotein. Biochemistry. 36: 11 298−303.
- Macfarlane J., Muller M. (1995) The functional integration of a polytopic membrane protein of Escherichia coli is dependent on the bacterial signal-recognition particle. Eur. J. Biochem. 233: 766−771.
- Manoil C. (1991) Analysis of membrane protein topology using alkaline phosphatase and P-galactosidase gene fusions. Methods Cell Biol. 34: 61−75.
- Manoil C., Mekalanos J.J., Beckwith J. (1985) TnphoA: a transposon probe for protein export signals. Proc. Natl. Acad. Sci. USA. 82: 8129−8133.
- Matsumoto G., Mori H., Ito K. (1998) Roles of SecG in ATP- and SecA-dependent protein translocation. Proc. Natl. Acad. Sci. USA. 95: 13 567−72.
- Matz M.V., Fradkov A.F., Labas Y.A., Savitsky A.P., Zaraisky A.G., Markelov M.L., Lukyanov S.A. (1999) Fluorescent proteins from nonbioluminescent Anthozoa species. Nat. Biotechnol. 17: 969−973.
- McGuffin L.J., Bryson K., Jones D.T. (2000) The PSIPRED protein structure prediction server. Bioinformatics. 16: 404−405.
- Miller J.D., Bernstein H.D., Walter P. (1994) Interaction of E. coli Ffh/4.5S ribonucleoprotein and FtsY mimics that of mammalian signal recognition particle and its receptor. Nature. 367: 657−659.
- Moller S., Croning M.D.R., Apweiler R. (2001) Evaluation of methods for the prediction of membrane spanning regions. Bioinformatics. 17: 646−653.
- Mori H., lto K. (2001) The Sec protein-translocation pathway. Trends Microbiol. 9: 494−500.
- Muller M., Klosgen R.B. (2005) The Tat pathway in bacteria and chloroplasts. Mol. Membr. Biol. 22: 113−121.
- Murphy C.K., Kalve V.I., Klebba P.E. (1990) Surface topology of the Escherichia coli K-12 ferric enterobactin receptor. J. Bacteriol. 172: 2736−746.
- Nagamori S., Smirnova I.N., Kaback H.R. (2004) Role of YidC in folding of polytopic membrane proteins. J. Cell Biol. 165: 53−62.
- Nandineni M.R., Gowrishankar J. (2004) Evidence for an arginine exporter encoded by yggA (argO) that is regulated by the LysR-Type Transcriptional Regulator ArgP in Escherichia coli. J. Bacteriol. 186: 3539−3546.
- Nilsson I.M., von Heijne G. J. (1993) Determination of the distance between the oligosaccharyltransferase active site and the endoplasmic reticulum membrane. Biol. Chcm. 268:5798−5801.
- Nishiyama K.I., Suzuki T., Tokuda H. (1996) Inversion of the membrane topology of SecG coupled with SecA-dependent preprotein translocation. Cell. 85: 71−81.
- Ogawa W., Kim Y-M., Mizushima T., Tsuchiya T. (1998) Cloning and expression of the gene for the Na±coupled serine transporter from Escherichia coli and characteristics of the transporter. J. Bacteriol. 180: 6749−6752.
- Oliver D.C., Paetzel M. (2008) Crystal structure of the major periplasmic domain of the bacterial membrane protein assembly facilitator YidC. J. Biol. Chem. 283: 52 085 216.
- Ormo M. B., Cubitt A., Kallio K., Gross L.A., Tsien R.Y., Remington S.J. (1996) Crystal structure of the Aequorea victoria green fluorescent protein. Science. 273:13 921 395.
- Palmieri L., Berns D., Kramer R., Eikmanns M. (1996) Threonine diffusion and threonine transport in Corynebacterium glutamicum and their role in threonine production. Arch. Microbiol. 165:48−54.
- Park J.H., Lee K.H., Kim T.Y., Lee S.Y. (2007) Metabolic engineering of Escherichia coli for the production of L-valine based on transcriptome analysis and in silico gene knockout simulation. PNAS. 104: 7797−7802.
- Peeters E., Le Minh P.N., Foulquie-Moreno M., Charlier D. (2009) Competitive activation of the Escherichia coli ArgO gene coding for an arginine exporter by the transcriptional regulators Lrp and ArgP. Mol. Microbiol: 74: 1513−1526.
- Peterson J.H., Woolhead C.A., Bernstein H.D. (2003) Basic amino acids in a distinct subset of signal peptides promote interaction with the signal recognition particle. J. Biol. Chem. 278: 46 155−46 162.
- Pittard J., Camakaris H., Yang J. (2005) The TyrR regulon. Mol. Microbiol. 55: 16−26.
- Pohlschroder M., Hartmann E., Hand N.J., Dilks K., Haddad A. (2005) Diversity and evolution of protein translocation. Annu. Rev. Microbiol. 59: 91−111.
- Polen T., Kramer M., Bongaerts J., Wubbolts M., Wendisch V.F. (2005) The global gene expression response of Escherichia coli to L-phenylalanine. J. Biotech. 115: 221−237.
- Pop O.I., Soprova Z., Koningstein G., Scheffers D.-J., van Ulsen P., Wickstrom
- D., de Gier J.-W., Luirink J. (2009) YidC is required for the assembly of the MscL homopentameric pore. FEBS J. 276: 4891−4899.
- Popov M., Tam L.Y., Li J., Reithmeier R.A. (1997) Mapping the ends of transmembrane segments in a polytopic membrane protein. Scanning N-glycosylation mutagenesis of extracytosolic loops in the anion exchanger, band 3. J. Biol. Chem. 272: 18 325−18 332.
- Poritz M.A., Bernstein H.D., Strub K., Zopf D., Wilhelm H., Walter P. (1990) An
- E. coli ribonucleoprotein containing 4.5S RNA resembles mammalian signal recognition particle. Science. 250: 1111−7.
- Pradel N., Decorps A.,'Ye C., Santini C.L., Wu L.F. (2005) YidC-dependent translocation of green fluorescence protein fused to the FliP cleavable signal peptide. Biochimie. 87: 191−6.
- Pradel N., Ye C., Wu L.F. (2004) A cleavable signal peptide is required for the full function of the polytopic inner membrane protein FliP of Escherichia coli. Biochem. Biophys. Res. Commun. 319: 1276−80.
- Price C.E., Driessen A.J.M. (2010) Conserved negative charges in the transmembrane segments of subunit K of the NADH: ubiquinone oxidoreductasedetermine its dependence on YidC for membrane insertion. J. Biol. Chem. 285: 35 753 581.
- Prinz W.A., Boyd D.H. Ehrmann M., Beckwith J. (1998) The protein translocation apparatus contributes to determining the topology of an integral membrane protein in Escherichia coli. 273: 8419−8424.
- Raine A., Uliers R., Pavlov M., Luirink J., Wikberg J.E., Ehrenberg M. (2003) Targeting and insertion of heterologous membrane proteins in E. coli. Biochimie. 85: 659−668.
- Rapoport T.A., Jungnickel B., Kutay U. (1996) Protein transport across the eukaryotic endoplasmic reticulum and bacterial inner membranes. Annu. Rev. Biochem. 65:271−303.
- Rapp M., Drew D., Daley D.O., Nilsson J., Carvalho T., Melen K., de Gier J.-W., von Heijne G. (2004) Experimentally based topology models for E. coli inner membrane proteins. Prot. Sei. 13: 937−945.
- Ravaud S., Stjepanovic G., Wild K., Sinning I. (2008) The crystal structure of the periplasmic domain of the Escherichia coli membrane protein insertase YidC contains a substrate binding cleft. J. Biol. Chem. 283: 9350−9358.
- Ribes V., Romisch K., Giner A., Dobberstein B., Tollervey D. (1990) E. coli 4.5S RNA is part of a ribonucleoprotein particle that has properties related to signal recognition particle. Cell. 63: 591−600.
- Rost B., Casadio R., Fariselli P., Sander C. (1995) Transmembrane helices predicted at 95% accuracy. Protein Sei. 4: 521−533.
- Rouanet C., Nasser W. (2001) The PecM protein of the phytopathogenic bacterium Erwinia chrysanthemi, membrane topology and possible involvement in the efflux of the blue pigment indigoidine. J. Mol. Microbiol. Biotechnol. 3: 309−318.
- Saier M.H. (1997) Multiple mechanisms controlling carbon metabolism in bacteria. Biotechnol. Bioeng. 58: 170−174.
- Sambrook J., Russell D.W. (2001) Molecular Cloning: Laboratory Mannual, 3rd edn. Cold Spring Harbor Laboratory Press, Cold Spring Harbor, New York.
- Samuelson J.C., Chen M., Jiang F., Moller I., Wiedmann M., Kuhn A., Phillips G.J., Dalbey R.E. (2000) YidC mediates both Sec-dependent and Sec-independent membrane protein insertion. Nature. 406: 637−641.
- Sanger F., Nicklen S., Coulson A.R. (1977) DNA sequencing with chain-terminating inhibitors. Proc. Natl. Acad. Sei. USA. 74: 5463−7.
- Schell M.A. (1993) Molecular biology of the LysR family of transcriptional regulators. Annu. Rev. Microbiol. 47: 597−626.
- Schoner R., Herrmann K.M. (1976) 3-Deoxy-D-arabino-heptulosonate 7-phosphate synthase. Purification, properties, and kinetics of the tyrosine-sensitive isoenzyme from Escherichia coli. J. Biol. Chem. 252: 5440−5447.
- Scotti P.A., Urbanus M.L., Brunner J., de Gier J.W., von Heijne G., van der Does C., Driessen A.J., Oudega B., Luirink J. (2000) YidC, the Escherichia coli homologue of mitochondrial Oxalp, is a component of the Sec translocase. EMBO J. 19: 542−549.
- Serek J., Bauer-Manz G., Struhalla G., van den Berg L., Kiefer D., Dalbey R., Kuhn A. (2004) Escherichia coli YidC is a membrane insertase for Sec-independent proteins. EMBO J. 23: 294−301.
- Shimomura O. (1979) Structure of the chromophore of Aequorea green fluorescent protein. FEBS Lett. 104: 220−222.
- Shimomura O., Johnson F.H., Saiga Y. (1962) Extraction, purification and properties of aequorin, a bioluminescent protein from the luminous hydromedusan, Aequorea. J. Cell. Comp. Physiol. 59: 223−239.
- Silhavy T.J., Benson S.A., Emr S.D. (1983) Mechanisms of protein localization. Microbiol. Rev. 47: 313−344.
- Simic P., Sahm H., Eggeling L. (2001) L-Threonine export: use of peptides to identify a new translocator from Corynebacterium glutamicum. J. Bacteriol. 183: 53 175 324.
- Snyder W.B., Silhavy T.J. (1995) (3-Galactosidase is inactivated by intermolecular disulfide bonds and is toxic when secreted to the periplasm of Escherichia coli. J. Bacteriol. 177: 953−963.
- Spira B., Silberstein N., Yagil E. (1995) Guanosine 3', 5'-bispyrophosphate (ppGpp) synthesis in cells of Escherichia coli starved for Pi. J. Bacteriol. 177: 40 534 058.
- Sumantran V.N., Schweizer H.P., Datta P.A. (1990) A membrane associated threonine permease encoded by the IcicC gene of Escherichia coli. J. Bacteriol. 172: 4288−4294.
- Tani T.H., Khodursky A., Blumenthal R.M., Brown P.O., Matthews R.G. (2002) Adaptation to famine: a family of stationary-phase genes revealed by microarray analysis. Proc. Natl. Acad. Sci. USA. 99: 13 471−13 476.
- Thornton J., Blakey D., Scanlon E., Merrick M. (2006) The ammonia channel protein AmtB from Escherichia coli is a polytopic membrane protein with a cleavable signal peptide. FEMS Microbiol. Lett. 258: 114−120.
- TrOtschel C., Deutenberg D" Bathe B., Burkovski A., Kramer R. (2005) Characterization of methionine export in Corynebacterium glutamicum. J. Bacteriol. 187: 3786−3794.
- Tsien R.Y. (1998) The green fluorescent protein. Annu. Rev. Biochem. 67: 509 544.
- Tusnady G.E., Simon I. (2001) The HMMTOP transmembrane topology prediction server. Bioinformatics. 17: 849−850.
- Typas A., Hengge R. (2006) Role of the spacer between the -35 and -10 regions in os promoter selectivity in Escherichia coli. Mol. Microbiol. 59: 1037−1051.
- Ubarretxena-Belandia I., Baldwin J.M., Schuldiner S., Tate C.G. (2003) Three-dimensional structure of the bacterial multidrug transporter EmrE shows it is an asymmetric homodimcr. EMBO J. 22: 6175−6181.
- Ulbrandt N.D., Newitt J.A., Bernstein H.D. (1997) The E. coli signal recognition particle is required for the insertion of a subset of inner membrane proteins. Cell. 88: 187−196.
- Urbanus M.L., Froderberg L., Drew D., Bjork P., de Gier J.-W., Brunner J., Oudega B., Luirink J. (2002) Targeting, insertion, and localization of Escherichia coli YidC. J. Biol. Chem. 277: 12 718−12 723.
- Valent Q.A., Kendall D.A., High S., Kusters R., Oudega B. and Luirink J. (1995) Early events in preprotein recognition in E. coli: interactions of SRP and trigger factoi with nascent polypeptides. EMBO J. 14: 5494−5505.
- Vrljic M., Kronemeyer W., Sahm H., Eggeling L. (1995) Unbalance of L-lysine flux in Corynebacteriurn glutamicum and its use for the isolation of excretion-defective mutants. J. Bacteriol. 177: 4021−4027.
- Vrljic M., Sahm H., Eggeling L. (1996) A new type of transporter with a new type of cellular function: L-lysine export from Corynebacteriurn glutamicum. Mol. Microbiol. 22: 815−826.
- Vrontou E., Economou A. (2004) Structure and function of SecA, the preprotein translocase nanomotor. Biochim. Biophys. Acta 1694: 67−80.
- Wagner S., Bader M.L., Drew D., de Gier J.-W. (2006) Rationalizing membrane protein overexpression. Trends Biotechnol. 24: 364−371.
- Wagner S., Pop O.I., Haan G.J., Baars L., Koningstein G., Klepsch M.M., Genevaux P., Luirink J., de Gier J.W. (2008) Biogenesis of MalF and the MalFGK (2) maltose transport complex in Escherichia coli requires YidC. J. Biol. Chem. 283: 1 788 190.
- Wang X., Bogdanov M., Dowhan W. (2002) Topology of polytopic membrane protein subdomains is dictated by membrane phospholipid composition. EMBO J. 21: 5673−5681.
- Ward W.W., Cormier M.J. (1979) An energy transfer protein in coelenterate bioluminescence. Characterization of the Renilla green-fluorescent protein. J. Biol. Chem. 254: 781−788.
- Weber W., Helms V., McCammon J., Langhoff P. (1999) Shedding light on the dark and weakly fluorescent states of green fluorescent proteins. Proc. Natl. Acad. Sci. USA. 96:6177−6182.
- Weiner J.H., Shaw G., Turner R.J., Trieber C.A. (1993) The topology of the anchor subunit of dimethyl sulfoxide reductase of Escherichia coli. J. Biol. Chem. 268: 3238−44.
- Xie K., Dalbey R.E. (2008) Inserting proteins into the bacterial' cytoplasmic membrane using the Sec and YidC translocases. Nat. Rev. Microbiol: 6: 234−244.
- Xie K., Kiefer D., Nagler G., Dalbey R.E., Kuhn A. (2006) Different regions of the nonconserved large periplasmic domain of Escherichia coli YidC are involved in the SecF interaction and membrane insertase activity. Biochemistry. 45: 13 401−13 408.
- Xiong X.F., Reznikoff W.S. (1993) Transcriptional slippage during the transcription initiation process at a mutant lac promoter in vivo. J.Mol. Biol. 231: 569−80.
- Yang F., Moss L.G., Phillips G.N. Jr. (1996) The molecular structure of green fluorescent protein. Nat. Biotechnol. 14: 1246−1251.
- Yang J., Ogawa Y., Camakaris H., Shimada T., Ishihama A., Pittard A.J. (2007) folA, a new member of the TyrR regulon in E. coli K-12. J. Bacteriol. 189: 6080−84.
- Yanushevich Y.G., Staroverov D.B., Savitsky A.P., Fradkov A.F., Gurskaya N.G., Bulina M.E., Lukyanov K.A. Lukyanov S.A. (2002) A strategy for the generation of non-aggregating mutants of Anthozoa fluorescent proteins. FEBS Lett. 511: 11−14.
- Yi L., Celebi N., Chen M., Dalbey R.E. (2004) Sec/SRP requirements and energetics of membrane insertion of subunits a, b, and c of the Escherichia coli FiFo ATP synthase. J. Biol. Chem. 279: 39 260−67.
- Yi L., Dalbey R.E. (2005) Oxal/Alb3/YidC system for insertion of membrane proteins in mitochondria, chloroplasts and bacteria. Mol. Membr. Biol. 22: 101−111.
- Yu Z., Koningstein G., Pop A., Luirink J. (2008) The conserved third transmembrane segment of YidC contacts nascent Escherichia coli inner membrane proteins. J. Biol. Chem. 283: 34 635−34 642.
- Yuan J., Zweers J.C., van Dijl J.M., Dalbey R.E. (2010) Protein transport across and into cell membranes in bacteria and archaea. Cell. Mol. Life Sci. 67: 179−199.
- Zakataeva N.P., Aleshin V.V., Tokmakova I.L., Troshin P.V., Livshits V.A. (1999) The novel transmembrane Escherichia coli proteins involved in the amino acid efflux. FEBS Lett. 452: 228−232.
- Zelazny A., Bibi E. (1996) Biogenesis and topology of integral membrane proteins: characterization of lactose permease-chloramphenicol acetyltransferase hybrids. Biochemistry. 35. 10 872−10 878.
- Zhang W., Bogdanov M., Pi J., Pittard A.J., Dowhan W. (2003) Reversible topological organization within a polytopic membrane protein is governed by a change in membrane phospholipid composition. J. Biol. Chem. 278: 50 128−50 135.
- Zittrich S., Kramer R. (1994) Quantitative discrimination of carrier-mediated excretion of isoleucine from uptake and diffusion in Corynebacteruim glutarnicum. J. Bacteriol. 176: 6892−6899.
- Закатаева Н.П., Кутукова Е. А., Гронский С. В., Трошин П. В., Лившиц В. А., Алешин В. В. (2006) Экспорт метаболитов белками семейства DMT и RhtB и их возможная роль в межклеточной коммуникации. Микробиология, Т.75, № 4, 509−20.
- Зубова Н.Н., Будавина А. Ю., Савицкий А. П. (2003) Спектральные и физико-химические свойства зеленого (GFP) и красного (drFP583) флуоресцирующих белков. Успехи биологической химии, Т.43, С.163−224.
- Каташкина Ж.И., Скороходова А. Ю., Зименков Д. В., Гулевич А. Ю., Минаева Н. И., Дорошенко В. Г., Бирюкова И. В., Машко С. В. (2005) НаправленноеIизменение уровня экспрессии генов в бактериальной хромосоме. Молекулярная биология, Т.39, С.823−831.
- Ковальская О.Н., Сергиев П. В., Богданов А. А., Донцова О. А. (2007) Структурно-функциональная анатомия сигналузнающей частицы: от бактерий до млекопитающих. Успехи биологической химии, Т.47, С. 129−188.
- Кутукова Е.А., Закатаева Н. П., Лившиц В. А. (2005) Экспрессия генов, кодирующих белки семейства RhtB, зависит от глобального регулятора Lrp. Молекулярная биология, Т.39, № 3, С. 374−378.
- Миллер Дж. (1976) Эксперименты в молекулярной генетике. Изд-во: Мир, -436с.
- Овчарова И.В., Еремина С. Ю., Миронов А. С. (2003) Определение функциональной значимости нуклеотидного состава в области старта транскрипции гена udp Escherichia coli. Генетика, Т.39, № 1, С. 1−9.