Развитие методов конструирования бесплазмидных рекомбинантных штаммов Escherichia coli: от Mu-зависимой «случайной» интеграции до сайт-специфических перестроек хромосомы
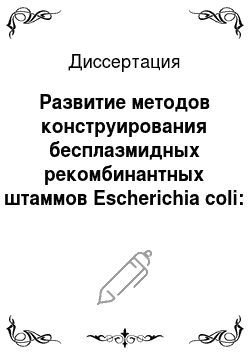
Диссертация
В настоящее время появляется все больше данных о том, что наличие в бактериальных клетках плазмид может негативно сказываться на жизнеспособности клеток, а именно: значительно изменять клеточный метаболизм, замедлять рост бактерий, что во многих случаях нежелательно при создании промышленных штаммов-продуцентов {Sauer U., 2001; Brownlie L. et al, 1990). Несомненный интерес к созданию нового… Читать ещё >
Список литературы
- Acroyd J., Symonds. 1983. Evidence for conservative pathway of transposition of bacteriophage Mw. Nature. 303, 84−86.
- Adler H. et al. 2000. Cloning and mutagenesis of the murine gammaherpesvirus 68 genome as an infectious bacterial artificial cromosome. J. Virol. 74, 6964−6974.
- Adzuma K., Mizuuchi K. 1988. Target immunity of Mu transposition reflects a differential distribution of MuB protein. Cell. 53, 257−266.
- Adzuma K., Mizuuchi K. 1991. Steady-state kinetic analysis of ATP hydrolysis by the В protein of bacteriophage Mu. J. Biol. Chem. 266, 6159−6167.
- Anderson D.G., Kowalczykowski S.C. 1997. The translocating RecBCD enzyme stimulates recombination by directing RecA protein onto ssDNA in a deregulated manner. Cell. 90, 77−86.
- Angrand P.-O., Daigle N., van der Hoeven F., Scholer H.R., Stewart A.F. 1999. Simplified generation of targeting constructs using ET recombination. Nucleic Acids Res. 27, el6.
- Arciszewska L.K., Sherratt D.J. 1995. Xer site-specific recombination in vitro. ENBOJ. 14,2112−2120.
- Arthur A., Sherratt D. 1979. Dissection of the transposition process: a transposon-encoded site-specific recombination system. Mol. Gen. Genet. 175, 267 274.
- Au Т.К., Agrawal P., Harshey R.M. 2006. Cromosomal integration mechanism of infecting Mu virion DNA. J. Bacteriology. 188, 1829−1834.
- Baba Т., Ara Т., Hasegawa M., Takai Y. et al. 2006. Construction of Escherichia coli K-12 in-frame, single-gene knockout mutants: the Keio collection. Molecular Systems Biology. 1−11.
- Bao Y., Lies D.P., Fu H., Roberts G.P. 1991. An improved Tn7-based system for the single-copy insertion of cloned genes into chromosomes of gram-negative bacteria. Gene. 109(1), 167−168.
- Barry G.F. 1988. A broad-host-range shuttle system for gene insertion into the chromosomes of Gram-negative bacteria. Gene. 71, 75−84.
- Baubonis W., Sauer B. 1993. Genomic targeting with purified Cre recombinase. Nucleic Acids Res. 21,2025−2029.
- Bernet A., Sabatier S., Picketts D.J., Ouazana R., Morle F., Higgs D.R. Godet J. 1995. Targeted inactivation of the major positive regulatory element (HS-40) of the human alpha-globin gene locus. Blood. 86, 1202−1211.
- Biswas T., Aihara H., Radman-Livaja M., Filman D., Landy A., Ellenberger T. 2005. A structural basis for allosteric control of DNA recombination by lambda integrase. Nature. 435, 1059−1066.
- Blakely G., Colloms S., May G., Burke M., Sherratt D. 1991. Escherichia coli XerC recombinase is required for chromosomal segregation at cell division. New Biol. 3(8), 789−798.
- Blakely G., May G., McCulloch R., Arciszewska L.K., Burke M., Lovett S.T., Sherratt DJ. 1993. Two related recombinases are required for site-specific recombination at dif and cer in E. coli K12. Cell. 75(2), 351−361.
- Blakely G.W., Sherratt D.J. 1994. Interactions of the site-specific recombinases XerC and XerD with the recombination site dif. Nucleic Acids Res. 22, 5613−5620.
- Blakely G.W., Davidson A.O., Sherratt D.J. 1997. Binding and cleavage of nicked substrates by site-specific recombinases XerC and XerD. J Mol Biol. 265(1), 30−39.
- Bloor A.E., Cranenburgh M.R. 2006. An efficient method of selectable marker gene excision by Xer recombination for gene replacement in bacterial chromosomes. Appl. and Enviroment. Microbiol. 72, 2520−2525.
- Bradford M.M. 1976. A rapid and sensitive method for the quantitationof microgram quantities of protein utilizing the principleof protein-dye binding. Anal Biochem. 72, 248−254.
- Brownlie L., Stephenson J.R., Cole J.A. 1990. Effect of growth rate on plasmid maintenance by Escherichia coli HB101(pAT153). J. Gen. Microbiol. 136, 24 712 480.
- Bruckner R.C., Cox M.M. 1986. Specific contacts between the FLP protein of the yeast 2-micron plasmid and its recombination site. J Biol Chem. 261, 1 179 811 807.
- Capaldo F.N., Barbour S.D. 1975. The role of the rec genes in the viability of Escherichia coli K12. Basic Life Sci. 5 A, 405−418.
- Castilho B.A., Casadaban M.J. 1991. Specifity of mini-Mu bacteriophage insertions in a small plasmid. J.Bacteriology. 173, 1339−1343.
- Chaconas G., Harshey R.M., Sarvetnick N., Bukhari A.I. 1981. Predominant end-products of prophage Mu DNA transposition during the lytic cycle are replicon fusions. J. Mol. Biol 150, 341−359.
- Chaconas G., Kennedy D.L., Evans D. 1983. Predominant end-products of infecting bacteriophage Mu DNA are simple insertions with no preference for integration of either Mu DNA strand. Virology. 128, 48 059.
- Chaconas G., Gloor G., Miller J.L. 1985. Amplification and purification of the bacteriophage Mu-encoded B transposition protein. J. Biol. Chem. 260, 2662−2669.
- Chaconas G., Lavoie B.D., Watson M.A. 1996. DNA transposition-jumping gene machine, some assembly required. Curr. Biol. 6, 817−820.
- Chen Y., Narenda U., Iype L.E., Cox M.M., Rice P.A. 2000. Crystal structure of a Flp recombinase-holliday junction complex: assembly of an active oligomer by helix swapping. Molecular Cell. 6, 885−897.
- Cherepanov P.P., Wackernagel W. 1995. Gene disruption in Escherichia coli: TcR and KmR cassettes with the option of Flp-catalyzed excision of the antibiotic-resistance determinant. Gene. 158, 9−14.
- Clark A.J. 1991. rec genes and homologous recombination proteins in Escherichia coli. Biochimie. 73, 523−532.
- Copeland N.G., Jenkins N.A., Court DL. 2001. Recombineering: a powerful new tool for mouse functional genomics. Nat Rev. 2, 769−779.
- Court D.L., Sawitzke J.A., Thomason L.C. 2002. Genetic engineering using homologous recombination. AnnuRev Genet. 36, 361−388.
- Cox M.M., Lehman I.R. 1987. Enzymes of general recombination. Annu. Rev. Biochem. 56, 229−262.
- Craig N.L. 1996. Transposition. Escherichia coli and Salmonella typhimurium: Cellular and Modular Biology. Eds Neidhart F.C. et al. Washington: Amer. Soc. Microbiol. 2, 2339−2362.
- Craigie R., Mizuuchi M., Mizuuchi K. 1984. Site-specific recognition of the bacteriophage Mu ends by the Mu A protein. Cell. 39, 387−394. •
- Dabert P., Smith G.R. 1997. Gene replacement with linear DNA fragments in wild-type Escherichia coli: enchancement by Chi sites. Genetics. 145, 877−889.
- Dale E.C., Ow D.W. 1991. Gene transfer with subsequent removal of the selection gene from the host genome. Proc. Natl. Acad. Sci. USA. 88, 10 558−10 562.
- Darzins A., Kent N.E., Buckwalter M.S., Casadaban M. J. 1988. Bacteriophage Mu sites required for transposition immunity. Proc. Natl. Acad. Sci. USA. 85, 68 266 830.
- Fukushige S., Sauer B. 1992. Genomic targeting with a positive-selection lox integration vector allows highly reproducible gene expression in mammalian cells. Proc. Natl. Acad. Sci. USA. 89, 7905−7909.
- Gerdes S.Y., Scholle M.D. et al. 2003. Experimental determination and system level analysis of essential genes in Escherichia coli MG1655. J. Bacteriology. 185, 5673−5684.
- Gopaul D. N, van Duyne G.D. 1999. Structure and mechanism in site-specific recombination. Current Opinion in Structural Biology. 9, 14−20.
- Grindley, N.D.F. 1994. Resolvase-mediated site-specific recombination. In: Nucleic Acids and Molecular Biology (ed. Eckstein F., Lilley D.M.J.). 236−267. Springer-Verlag, Berlin.
- Grindley, N.D.F. 1997. Site-specific recombination: synapsis and strand exchange revealed. Curr Biol. 7, R608—R612.
- Grindley N.D.F., Whiteson K.L., Rice P.A. 2006. Mechanisms of site-specific recombination. Annu. Rev. Biochem. 75, 567−605.
- Groisman E.A., Casadaban M.J. 1987. Cloning of genes from members of family Enterobacteriaceae with mini-Mu bacteriophage containing plasmid replicons. J. Bacteriology. 169, 687−693.
- Groth A.C., Calos M.P. 2004. Phage integrases: biology and application. J. Mol. Biol. 335(3), 667−678.
- Gueguen E., Rousseau P., Duval-Valentin G., Chandler M. 2005. The transpososome: control of transposition at the level of catalysis. Trends in Microbiology. 13, 543−549.
- Haapa S., Taira S., Heikkinen E., Savilahti H. 1999. An efficient and accurate integration of mini-Mu transposons in vitro: a general methodology for functional genetic analysis and molecular biology application. Nucleic Acids Research. 27, 2777−2784.
- Hayes F., Sherratt D.J. 1997. Recombinase binding specificity at the chromosome dimer resolution site dif of Escherichia coli. J. Mol. Biol. 266(3), 525 537.
- Haldimann A., Wanner B.L. 2001. Conditional-replication, integration, excision, and retrieval plasmid-host systems for gene structure-function studies of bacteria. JBacteriol. 183, 6384−6393.
- Hallet B., Sherratt D.J. 1997. Transposition and site-specific recombination: adapting DNA cut-and-past mechanism to a variety of genetic rearrangements. FEMS Microbiology Reviews. 21, 157−178.
- Hamilton C.M., Aldea M., Washburn B.K., Babitzke P., Kushner S.R. 1989. New method for generating deletions and gene replacements in Escherichia coli. J. Bacteriology. 171, 4617−4622.
- Harshey R.M., Getzoff E.D., Baldwin D.L. 1985. Primary structure of the phage Mu transposase: homology to Mu repressor. Proc. Natl. Acad. Sci. USA. 82, 76 767 680.
- Herrero M., de Lorenzo V., Timmis K.N. 1990. Transposon vectors containingnon-antibiotic resistance selection markers for cloningand stable chromosomal insertion of foreign genes in gram-negative bacteria. J Bacteriol. 172, 6557−6567.
- Hill F. et al. 2000. BAC trimming: minimizing clone overlaps. Genomics. 64, 111−113.
- Hoess R.H., Ziese M., Sternberg N. 1982. PI site-specific recombination: nucleotide sequence of the recombining sites. Proc. Natl Acad. Sci. USA. 79, 33 983 402.
- Hoess R.H., Abremski K. 1984. Interaction of the bacteriophage PI recombinase Cre with the recombining site loxP. Proc. Natl Acad. Sci. USA. 81, 1026−1029.
- Hoess R.H., Wierzbicki A., Abremski K. 1986. The role of the loxP spacer region in PI site-specific recombination. Nucleic Acids Res. 14, 2287−2300.
- Hsu P.-L., Ross W., Landy A. 1980. The Xphage att site: functional limits and interaction with Int protein. Nature. 285, 85−91.
- Huang L.C., Wood E.A., Cox M.M. 1991. A bacterial model system for chromosomal targeting. Nucleic Acids Res. 19, 443−448.
- Huang L.C., Wood E.A., Cox M.M. 1997. Convenient and reversible sitespecific targeting of exogenous DNA into a bacterial chromosome by use of the FLP recombinase: the FLIRT system. J Bacteriol. 179, 6076−6083.
- Johnson R.C. 1991. Mechanism of site-specific DNA inversion in bacteria. Curr. Opin. Genet. Dev. 1, 404−411.
- Johonson R.C. 1995. Site-specific recombinases an theire interaction with DNA. In: DNA-protein: structural interactions (ed. Lilley D.M.J.). 141−176. IRL Oxford.
- Joseph J.W., Kolodner R. 1983. Exonuclease VIII of Escherichia coli. Purification and physical properties. J.Biol. Chem. 258, 10 411−10 417.
- Karakousis G., Ye N., Li Z, Chiu S.K., Reddy G., Radding C.M. 1998. The beta protein ofphage lambda binds preferentially to an intermediate in DNA renaturation. J. Mol. Biol 276, 721−731.
- Kato C., Ohmiya R., Mizuno T. 1998. A rapid method for disrupting genes in the Escherichia coli genome. Biosci. Biotechnol. Biochem. 62(9), 1826−1829.
- Kikuchi Y., Tsujimoto K., Kurahashi O. 1997. Mutational analysis of the feedback sites of phenylalanine-sensitive 3-deoxy-D-arabino-heptulosonate-7-phosphate synthase of Escherichia coli. App. I Environ. Microbiol. 63, 761−762.
- Kobryn K., Lavoie B.D., Chaconas G. 1999. Supercoiling-dependent site-specific binding of HU to naked Mu DNA. J. Mol. Biol. 289, 777−784.
- Kolodner R. et al. 1994. Homologous pairing proteins encoded by the Escherichia coli recE and recTgenes. Mol. Microbiol. 11, 23−30.
- Komoda Y., Enomoto M., Tominaga A. 1991. Large inversion in Escherichia coli K-12 1485IN between inversely oriented IS3 elements near lac and cdd. Genetics. 129, 639−645.
- Kowalczykowski S.C., Dixon D.A., Eggleston A.K., Lauder S.D., Rehrauer W.M. 1994. Biochemistry of homologous recombination in Escherichia coli. Microbiol. Rev. 58, 401−465.
- Kulkarni S.K., Stahl F.W. 1989. Interaction between the sbcC gene of Escherichia coli and the gam gene of phage lambda. Genetics. 123, 249−253.
- Kuo C., Zou A., Jayaram M. 1991. A DNA-protein complex during attachmentsite synapses in Mu DNA transposition. EMBOJ. 10, 1585−1591.
- Kushner S.R., Nagaishi H., Clark A.J. 1974. Isolation of exonuclease VIII: the enzyme associated with the sbcA indirect supressor. Proc. Natl. Acad. Sci. USA. 71, 3593−3597.
- Landy A. 1989. Dynamic, structural, and regulatory aspects of lambda site-specific recombination. Annu Rev Biochem. 58, 913−949.
- Landy A. 1993. Mechanistic and structural complexity in the site-specific recombination pathway of Int and FLP. Curr. Opin. Genet. Dev. 3, 699−707.
- Lamberg A., Nieminen S., Qiao M., Savilahti H. 2002. Efficient insertion mutagenesis strategy for bacterial genomes involving electroporation of in vitro-assembled DNA transposition complexes of bacteriophage Mu. Appl. Envirom. Microbiol. 68, 705−712.
- Lavoie B.D., Chan B.S., Allison R.G., Chaconas G. 1991. Structural aspects of a higher order nucleoprotein complex: induction of an altered DNA structure at the Mu-host junction of the Mu type 1 transpososome. EMBOJ. 10, 3051−3059.
- Lavoie B.D., Chaconas G. 1996. Transposition of phage Mu DNA. Curr. Top. Microbiol. Immunol. 204, 83−102.
- Lee E.C., Gumport R.I., Gardner J.F. 1990. Genetic analysis of bacteriophage A, integrase interactions with arm-type attachment site sequences. J.Bacteriology. 172, 1529−1538.
- Lee J., Jayaram M. 1993. Mechanism of site-specific recombination. J. Biological Chemistry. 268, 17 564−17 579.
- Lee G., Saito I. 1998. Role of nucleotide sequences of loxP spacer region in Cre-mediated recombination. Gene. 216, 55−65.
- Leslie N.R., Sherratt D.J. 1995. Site-specific recombination in the replication terminus region of Escherichia coli: functional replacement of dif. EMBO J. 14, 1561−1570.
- Leung P.C., Teplow D.W., Harshey R.M. 1989. Interaction of distinct domains in Mu transposase with Mu DNA ends and an internal transpositional enhancer. Nature. 338, 656−658.
- Levchenko I., Yamauchi M., Baker T.A. 1997. ClpX and MuB interact with overlapping regions of Mu transposase: implications for control of the transposition pathway. Genes Dev. 11, 1561−1572.
- Li Z., Karakousis G., Chiu S.K., Reddy G., Radding C.M. 1998. The beta protein of phage lambda promotes strand exchange. J. Mol. Biol. 276, 733−744.
- Liebart J.C., Ghelardini P., Paolozzi L. 1982. Conservative integration of bacteriophage Mu DNA into pBR322 plasmid. Proc. Natl. Acad. Sci. USA. 79, 43 624 366.
- Lloyd R.G., Barbour S.D. 1974. The genetic location of the sbcA gene of Escherichia coli. Mol. Gen. Genet. 134, 157−171.
- Lloyd R.G., Buckman C. 1985. Identification and genetic analysis of sbcC mutations in commonly used recBC sbcB strains of Escherichia coli K-12. J. Bacteriology. 164, 836−844.
- Luetke K.H., Zhao B.P., Sadowski P.D. 1997. Asymmetry in Flp-mediated cleavage. Nucleic Acids Res. 25(21): 4240−4249.
- Luetke K.H., Sadowski P.D. 1998. DNA sequence determinant for Flp-induced DNA bending. Mol. Microbiol. 29, 199−208.
- MacWfiams M.P., Gumport R.I., Garher J.F. 1996. Genetic analysis of the bacteriophage X attL nucleoprotein complex. Genetics. 143, 1069−1079.
- Mac Williams M., Gumport R.I., and Gardner J.F. 1997. Mutational analysis of protein binding sites involved in formation of the bacteriophage lambda attL complex. JBacteriol. 179(4), 1059−1067.
- Marinus M.G., Carraway M., Frey A.Z., Brown L., Arraj J.A. 1983. Insertion mutations in the dam gene of Escherichia coli K-12. Mol. Gen. Genet. 192, 288−289.
- Marsic N., Roje S., Stojiljkovic I., Salaj-Smic E., Trgovcevic Z. 1993. In vivo studies on the interaction of RecBCD enzyme and lambda Gam protein. J. Bacteriology. 175, 4738−4743.
- Matsuura S., Komatsu J., Hirano K., Yasuda H., Takashima K. et al. 2001. Realtime observation of a single DNA digestion by X exonuclease under a fluorescence microscope field. Nucleic Acids Res. 29, E79.
- McClain M.S., Blomfield I.C., Eisestein B.I. 1991. Roles of fimB and fimE insite-specific DNA inversion associated with phase variation of type 1 fimbriae in Escherichia coli. JBacteriol. 173, 5308−5314.
- McKenzie G.J., Craig N.L. 2006. Fast, easy and efficient: site-specific insertion of transgenes into Enterobacterial chromosomes using Tn7 without need for selection of the insertion event. BMC Microbiology. 6:39.
- Medberry S.L., Dale E., Qin M., Ow. D.W. 1995. Intra-chromosomal rearrangements generated by Cre-lox site-specific recombination. Nucleic Acids Res. 23, 485−490.
- Messerle M. et al. 1997. Cloning and mutagenesis of a herpesvirus genome as an infectious bacterial artificial cromosome. Proc. Natl. Acad. Sei. USA. 94, 1 475 914 763.
- Meynial-Salles I., Cervin M.A., Soucaille P. 2005. New tool for metabolic pathway engineering in Escherichia coli: one-step method to modulate expression of chromosomal genes. Applied and Enviromental Microbiology. 71, 2140−2144.
- Miller J.H. 1972. Experiments in molecular genetics, Cold Spring Harbor, N.Y.: Cold Spring Harbor Lab. Press.
- Mizuuchi K., Craigie R. 1986. Mechanism of bacteriophage Mu transposition. Ann. Rev. Genet. 20, 385−429.
- Mizuuchi M., Mizuuchi K. 1989. Efficient Mu transposition requires interaction of transposase with a DNA sequence at the Mu operator: implications for regulation. Cell. 58, 399−408.
- Mizuuchi M., Baker T.A., Mizuuchi K. 1991. DNase protection analysis of the stable synaptic complexes involved in Mu transposition. Proc. Natl. Acad. Sci. USA. 88, 9031−9035.
- Mizuuchi K. 1992. Polynucleotidyl transfer reactions in transpositional DNA recombination. J. Biol. Chem. 267, 21 273−21 276.
- Mizuuchi K. 1992. Transpositional recombination: mechanistic insights from studies of Mu and others elements. Annu. Rev. Biochem. 61, 1011−1051.
- Muniyappa K., Radding C.M. 1986. The homologous recombination system of phage lambda. Pairing activities of beta protein. J. Biol. Chem. 261, 7472−7478.
- Murphy K.C. 1998. Use of bacteriophage X recombination functions to promote gene replacement in Escherichia coli. JBacteriol. 180, 2063−2071.
- Murphy K.C., Campellone K.G., Poteete A.R. 2000. PCR-mediated gene replacement in Escherichia coli. Gene. 246, 321−330.
- Muyrers J.P.P., Zhang Y., Testa G., Stewart A.F. 1999. Rapid modification of bacterial artificial chromosomes by ET-recombination. Nucleic Acids Res. 27, 15 551 557.
- Muyrers J.P.P. et al. 2000. Point mutation of bacterial artificial chromosomes by ET recombination. EMBO Rep. 1, 239−243.
- Muyrers J.P.P., Zhang Y., Stewart A.F. 2000. ET-cloning- think recombination first. In Genetic engineering. 22 (ed. Setlow J.K.), 77−98.
- Muyrers J.P.P., Zhang Y., Buchholz F., Stewart A.F. 2000. RecE/RecT and Reda/Redp initiate double stranded break repair by specofocally interacting with their respective partners. Genes Dev. 14, 1971−1982.
- Muyrers J.P.P., Zhang Y., Stewart A.F. 2001. Techniques: recombinogenic engineering new options for cloning and manipulating DNA. Trends in Biochemical Sciences. 26, 325−331.
- Myers R.S., Stahi F.W. 1994. % and RecBCD enzyme of Escherichia coli. Annu. Rev. Genet. 28, 49−70.
- Mythili E., Kumar K.A., Muniyappa K. 1996. Characterization of the DNA-binding domain of beta protein, a component of phage lambda red-pathway, by UV catalyzed crosslinking. Gene. 182, 81−87.
- Naigamwalla D.Z., Chaconas G. 1997. A new set of Mu DNA transposition intermediates: alternate pathways of target capture preceding strand transfer. EMBO J. 16, 5227−5234.
- Nakai H., Doseeva V., Jones J.M. 2001. Handoff from recombinase to replisome: insights from transposition. PNAS. 98, 8247−8254.
- Nefedov M., Williamson R., Ioannou P.A. 2000. Insertion of disease-causing mutations in BACs by homologous recombination in Escherichia coli. Nucleic Acids Res. 28, E79.
- Ogawa T.H., Wabiko H., Tsurimoto T., Horii T., Masukata H., Ogawa H. 1979. Characteristics of purified recA protein and the regulation of its synthesis in vivo. Cold Spring Harbor Symp. Quant. Biol. 43, 909−915.
- Payne C.M. et al. 1999. Manipulating large genomic clones via in vivo recombination in bacteria. J. Hum. Hypertens. 13, 845−848.
- Peredelchuk M.Y., Bennet G.N. 1997. A method for construction of E. coli strains with multiple DNA insertions in the chromosome. Gene. 187(2), 231−238.
- Posfai G., Koob M., Hradecna Z., Hasan N., Filutowicz M., Szybalski W. 1994. In vivo excision and amplification of large segments of the Escherichia coli genome. Nucl. Acids Res. 22(12), 2392−2398.
- Posfai G., Kolisnychenko V., Bereczki Z., Blattner F.R. 1999. Markerless gene replacement in Escherichia coli stimulated by a double-strand break in the chromosome. Nucleic Acids Res. 27, 4409−4415.
- Poteete A.R., Fenton A.C. 2000. Genetic requirments of phage X Red-mediated gene replacement in Escherichia coli K12. J. Bacteriology. 182, 2336−2340.
- Poustka A. et al. 1984. Selective isolation of cosmid clones by homologous recombination in Escherichia coli. Proc. Natl. Acad. Sci. USA. 81, 4129−4133.
- Radding C.M. 1982. Homologous pairing and strand exchange in genetic recombination. Annu. Rev. Genet. 16, 405−437.
- Ramirez S.R., Liu P., Bradley A. 1995. Chromosome engineering in mice. Nature. 378, 720−724.
- Roland L.A.S., Baker T.A. 2001. Differential role of the MuB protein in phage Mu integration vs. replication: mechanistic insights into two transposition pathways. Mol. Microbiol. 40(1), 141−155.
- Ross W., Landy A., Kikuchi Y., Nash H. 1979. Interaction of int protein with specific sites on lambda art DNA. Cell. 18, 297−307.
- Russell C.B., Thaler D.S., Dahlquist F.W. 1989. Chromosomal transformation of Escherichia coli recD strains with linearized plasmids. J. Bacteriology. 171, 26 092 613.
- Sadowski P.D. 1993. Site-specific genetic recombination: hops, flips, and flops. FASEB J. 7, 760−767.
- Sadowski P.D. 1995. The Flp recombinase of the 2-microns plasmid of Saccharomyces cerevisiae. Prog Nucleic Acid Res Mol Biol. 51, 53−91.
- Salaj-Smic E., Dermic D., Brcic-Kostic K., Cajo G.C., Trgovcevic E. 2000. In vivo studies of the Escherichia coli RecB polypeptide lacking its nuclease center. Res. Microbiol. 151, 769−776.
- Sambrook J., Fitsch E.F., Maniatis T. Molecular Cloning: A Laboratory Manual Cold Spring Harbor, Cold Spring Harbor Press- 1989.
- Sauer B. 1994. Site-specific recombination: developments and applications. Curr. Opin. Biotechnol. 5, 521—527.
- Sauer U. 2001. Evolutionary engineering of industrially important microbial phenotypes. Adv. Biochem. Eng Biotechnol. 73, 129−169.
- Schofield M.A., Agbunag R., Miller J.H. 1992. DNA inversions between short inverted repeats in Escherichia coli. Genetics. 132, 295−302.
- Senecoff J.F., Bruckner R.C., Cox M. M. 1985. The FLP recombinase of the yeast 2-micron plasmid: characterization of its recombination site. Proc. Natl. Acad. Sci. USA.82, 7270−7274.
- Shapiro J.A. 1979. Molecular model for the transposition and replication of bacteriophage Mu and other transposable elements. Proc. Natl. Acad. Sci. USA. 76, 1933−1937.
- Sherrat, D.J. 1995. Mobile genetic elements. Oxford University Press, Oxford, England.
- Smith G.R. 1988. Homologous recombination in procaryores. Microbiological reviews. 52, 1−28.
- Smith G.R. 1989.. Homologous recombination in E. coli: multiple pathways for multiple reasons. Cell. 58, 807−809.
- Smith G.R. 1994. Hotspots of homologous recombination. Experientia. 50, 234 241.
- Smith M.C., Thorpe H.M. 2002. Diversity in the serine recombinases. Mol.Microbiol. 44, 299−307.
- Sousa C., de Lorenzo V., Cebolla A. 1997. Modulation of gene expression through chromosomal positioning in Escherichia coli. Microbiology. 143, 20 712 078.
- Stark W.M., Boocock M.R. 1995. Topological selectivity in site-specific recombination. In: Mobil Genetic Elements (ed. Sherratt D.J.). 101−129. IRL Oxford.
- Sternberg N. 1990. Bacteriophage PI cloning system for the isolation, amplification and recovery of DNA fragments as large as 100 kilobase pairs. Proc Natl Acad Sci USA. 87, 103−107.
- Surette M.G., Lavoie B.D., Chaconas G. 1989. Action at a distance in Mu DNA transposition: an enhancer-like element is the site of action of supercoiling relief activity by IHF. EMBOJ. 8, 3483−3489.
- Surette M.G., Chaconas G. 1989. A protein factor that reduces the negative supercoiling requirement in the Mu strand-transfer reaction is Escherichia coli integration host factor. J. Biol. Chem. 264, 3028−3034.
- Taylor A.F., Schultz D.W., Ponticelli A.S., Smith G.R.1985. RecBC enzyme nicking at Chi sites during DNA unwinding: location and orientation dependence of the cutting. Cell. 41, 153−163.
- Telander-Muskavitch K.M., Linn S. 1981. RecBC-like enzymes: the exonuclease V deoxyribonucleases. 233−250. In P.D. Boyer (ed.). The enzymes, vol. 14. Academic Press. Inc., New York.
- Van Duyne G.D. 2001. A structural view of cre-loxp site-specific recombination. Annu Rev Biophys Biomol Struct. 30, 87−104.
- Van Gijsegem F., Toussaint A., Casadaban M. 1987. Mu as genetic tool. Phage Mu. Edited by Symonds N. chapter 14, 215−250.
- Van de Putte P., Goosen N. 1992. DNA inversion in phage and bacteria. Trends Genet. 8, 457−462.
- Wang В., Liu L., Groisman E.A., Casadaban M.J., Berg C.M. 1987. High frequency generalized transduction by miniMu plasmid phage. Genetics. 116, 201 206.
- Wang X., Higgins N.P. 1994. «Muprints» of the lac operon demonstrate physiological control over the randomness of in vivo transposition. Mol. Microbiol 12, 665−677.
- Yang X.W. et al. 1997. Homologous recombination based modification in Escherichia coli and germline transmission in transgenic mice of a bacterial artificial chromosome. Nat. Biotechnol. 15, 859−865.
- Yu В .J., Sung B.H., Koob M.D., Lee C. H, Lee J.H., Lee W. S, Kim M.S., Kim S.C. 2002. Minimization of the Escherichia coli genome using a Tn5-targeted Crq/IoxP excision system. Nature Biotechnol. 20, 1018−1023.
- Yu D., Ellis H.M., Lee E.C., Jenkins N.A., Copeland N.G., Court D.L. 2000. An efficient recombination system for chromosome engineering in Escherichia coli. Proc Natl Acad Sci USA. 97, 5978−5983.
- Zhang Y., Buchholtz F., Muyrers J.P.P., Stewart A.F. 1998. A new logic forDNA engineering using recombination in Escherichia coli. Nature Genetics. 20, 123−128.
- Zaman M.M., Boles T.C. 1996. Plasmid recombination by the RecBCD pathway of Escherichia coli. J. Bacteriology. 178, 3840−3845.
- Zhang Y., Muyrers J.P.P., Testa G., Stewart A.F. 2000. DNA cloning by homologous recombination in Escherichia coli. Nature Biotechnology. 18, 13 141 317.
- Zhou J.G., Hong X., Huang C.F. 2003. Recombineering and its application. Yi Chuan Xue Bao. 30, 983−988. Zhou J.G., Hong X., Huang C.F. 2003. Recombineering and its application. Yi Chuan Xue Bao. 30, 983−988.
- Каташкина Ж.И., Скороходова А. Ю., Зименков Д. В., Гулевич А. Ю., Минаева Н. И., Дорошенко В. Г., Бирюкова И. В., Машко С. В. 2005. Направленное изменение уровня экспрессии генов в бактериальной хромосоме. Молекулярная биология. 39, 823−831.
- Митькина JI.H. 2003. Транспозиция как способ существования:-фаг Ми. Генетика. 5, 637−656.